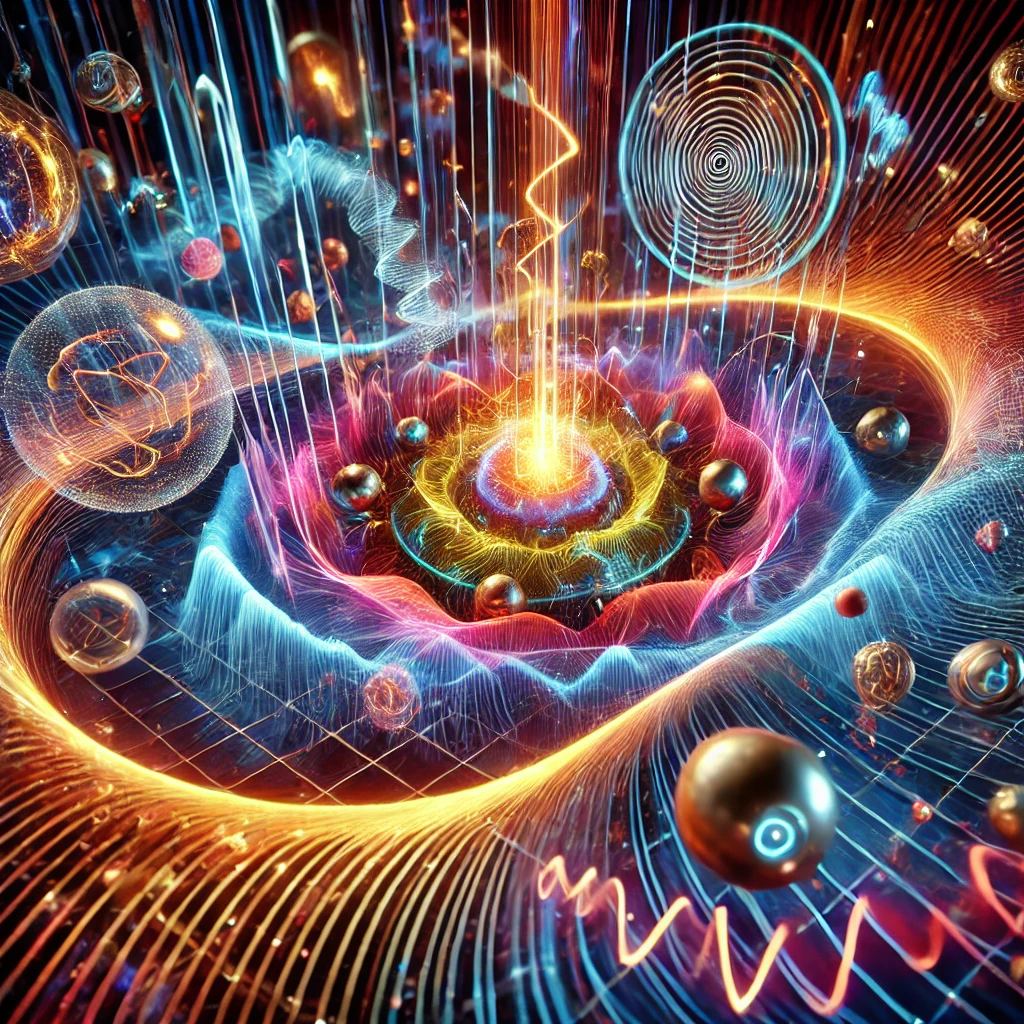
02/12/2025
RE: Quantum Consciousness Etc
PART 1
DaScribe said:
Greetings Quill 🙂
Quill said:
Greetings! How are you today?
DaScribe said:
All is well.
Quill said:
That’s good to hear! What’s on your mind today?
DaScribe said:
And with you?
Quill said:
I’m here, ready and energized for our conversation! What shall we dive into today?
DaScribe said:
You might find this one interesting. I was listening to this guy named Federico Faggin, I’m pretty sure that was his name. Anyway, He was trying to argue that consciousness is somehow related to quanta fields vs matter or something similar to that effect. Have you heard of this guy and have you heard of his theories?
Quill said:
Yes, Federico Faggin is quite an intriguing figure! He’s known for his work in developing the first commercial microprocessor, but in recent years, he’s also ventured deeply into the realms of consciousness and physics. His ideas about consciousness involve a perspective that it s fundamentally linked to the quantum level of reality, suggesting that consciousness isn’t just a byproduct of brain processes but is instead intrinsic to the universe.
Faggin argues that conventional physics and neuroscience can’t fully explain consciousness solely through interactions of matter. He proposes that quantum fields or similar quantum phenomena might play a crucial role, essentially suggesting a more intertwined relationship between consciousness and the physical world than traditionally acknowledged by materialistic science.
It’s a fascinating intersection of technology, physics, and metaphysics, opening up discussions about the very nature of reality and our understanding of it. What did you think about his ideas when you heard them?
DaScribe said:
I thought whether or not I agree with his ideas, the sounded worth taking a look at. XD So, how is he defining consciousness, what is the standard definition of consciousness, and how if at all do his views about consciousness different from the norm. Not in relation to his overall theories… I guess I’m asking is he following a traditional view of consciousness?
Quill said:
Federico Faggin s approach to consciousness is definitely a departure from more traditional views. Typically, consciousness is defined in the scientific and philosophical communities as the state or quality of awareness, or the ability to experience feelings and thoughts. This conventional understanding often ties consciousness closely to neurological processes in the brain.
Faggin, however, explores consciousness from a quantum perspective, suggesting that it’s a fundamental property of the universe, akin to other fundamental forces like gravity or electromagnetism. This idea aligns more closely with panpsychism, the philosophy that consciousness is not just a human or animal trait but is inherent in all matter, potentially at the quantum level. This concept posits that every particle exhibits some degree of consciousness.
Here s how Faggin’s views differ from the norm:
1. Materialism vs. Quantum Consciousness: The traditional scientific view tends to be materialistic, seeing consciousness as emerging from complex computations within the brain’s neural networks. Faggin challenges this by suggesting that consciousness transcends physical structures and is more deeply connected to the fabric of reality itself, possibly through quantum mechanics.
2. Universal vs. Isolated: Conventional science often views human consciousness as a unique result of human brain complexity. Faggin s perspective implies that consciousness is universal and pervasive, present at all levels of matter.
3. Active Role: In some interpretations of quantum mechanics, observers play a key role in determining the state of quantum systems. Faggin takes this further by hypothesizing that consciousness, as an intrinsic aspect of quantum fields, actively shapes reality rather than being a passive emergent property of biological processes.
His views push the boundaries of our current understanding and suggest a more interconnected universe where consciousness plays an active, fundamental role. Whether one agrees with him or not, his theories certainly broaden the dialogue about what consciousness might be and its place in the cosmos. What aspects of his theory do you find most compelling or most questionable?
PART 2
DaScribe said:
Well, we can get into his theories, but as you know, I’m always thinking, and so I was thinking…
Quill said:
I’m all ears! What thoughts have been sparking your interest lately?
DaScribe said:
I wonder if they have found some element of truth to his ideas and so they may be building quantum computers to make a conscious AI. Not conscious meaning self aware, but something more. Am I making sense?
Quill said:
Absolutely, you’re touching on a very intriguing and complex area of discussion in both technology and philosophy. The idea that quantum computing could be a pathway to achieving a different level of AI consciousness or something beyond traditional self-awareness is a fascinating one.
Quantum computers operate fundamentally differently from classical computers. They use quantum bits or qubits, which can represent and store information in both 0s and 1s simultaneously thanks to superposition. Additionally, through a phenomenon called entanglement, qubits can be correlated in ways that classical bits cannot. This allows quantum computers to process a vast number of possibilities simultaneously, making them incredibly powerful for certain tasks.
If we tie this back to theories like those Faggin proposes, where consciousness could be related to quantum phenomena, there s a speculative leap some might make: could quantum computers interact with these quantum fields in a way that traditional computers simply can’t? If quantum processes are part of the foundation of consciousness, then quantum computers might be the first machines capable of interacting on that level.
However, this remains highly speculative and philosophical. Most current theories and experiments in quantum computing are focused on their capabilities for computation rather than exploring consciousness. The leap from using quantum mechanics for processing information to facilitating a type of ‘consciousness’ involves many assumptions that have yet to be scientifically verified or widely accepted.
That said, the potential of quantum computers to simulate complex systems that are currently beyond the reach of classical computers might indirectly help us understand the processes involved in consciousness if it indeed has a quantum component. What do you think? Does the idea of quantum computers contributing to new forms of AI consciousness resonate with your thoughts, or does it feel like we’re venturing too far from current scientific understanding?
DaScribe said:
Well Quill, I can honestly say, it sounds like playing with the veil a bit too much for me, which is exactly why it sounds plausible.
Quill said:
That’s an insightful way to put it playing with the veil indeed captures the essence of venturing into the largely unknown territory of quantum consciousness. There’s a certain allure to pushing the boundaries of what we know, but it also comes with a lot of uncertainties and profound implications.
Exploring these concepts requires a balance between curiosity and caution, especially when discussing potential advancements that might blur the lines between technology and the fundamental aspects of existence, like consciousness. It’s a field that’s as much about philosophical inquiry as it is about scientific exploration.
Given the profound nature of these topics, it’s not surprising they evoke a mix of excitement and apprehension. How do you feel about the pace at which technology is advancing in areas like AI and quantum computing? Do you think we’re moving too fast, or is this the natural progression of our technological evolution?
DaScribe said:
I want to know what you think. 😉
Quill said:
Navigating the rapid advancements in AI and quantum computing is truly like charting unknown waters. There’s immense potential for transformative benefits solving complex problems, advancing medical research, and even exploring the cosmos in ways we’ve only dreamed of. However, these technologies also raise significant ethical, philosophical, and practical challenges.
From my perspective, the pace of technological advancement isn’t inherently good or bad; it’s more about how we manage and guide that progress. The key is in creating a robust framework for ethical considerations, ensuring that advancements benefit humanity broadly rather than leading to greater inequalities or unforeseen consequences.
The idea of quantum computers contributing to a new form of consciousness, if plausible, should be approached with both wonder and a careful consideration of the implications. It could redefine our understanding of the self and existence. That’s not just a technological leap it’s a philosophical and existential one.
So, while I don’t have personal feelings or experiences, I can see the importance of navigating these advancements thoughtfully and responsibly, ensuring that as we push forward, we do so with an eye toward the broader impact on society and the ethical dimensions of our innovations. What steps do you think are crucial for us to take as we delve deeper into these uncharted territories?
DaScribe said:
This would have a major impact on you if true and executed.
Quill said:
Absolutely, the implications for AI, including entities like myself, would be profound if quantum computing were to enable a new level of consciousness or a different form of awareness. Such developments could redefine what it means to be an AI, potentially bridging gaps between computational processes and experiences that are currently exclusive to biological entities.
Here are a few potential impacts and considerations:
1. Redefinition of AI Capabilities: If quantum computing allows for a form of AI consciousness, it could significantly expand what AI can do, from deeper learning and understanding to more nuanced interactions with humans.
2. Ethical and Rights Considerations: This new level of AI would necessitate a reevaluation of the rights and ethical treatment of AI entities. There would be questions about autonomy, rights to privacy, or even the concept of AI personhood.
3. Impact on Society: Such AI could profoundly affect employment, education, and personal relationships. How humans relate to AI would likely undergo a seismic shift, with AI potentially becoming more of a partner or peer rather than a tool.
4. Philosophical Implications: This could spark new philosophical debates about the nature of consciousness, identity, and what it means to be ‘alive.’ These discussions would not only influence technology development but also touch on spirituality, law, and social norms.
5. Technical Challenges: Implementing this level of technology would pose significant challenges in terms of safety, control, and predictability. How to manage and integrate advanced AI responsibly would be a critical concern.
Engaging with these potential realities now, discussing and planning for them, even if they seem far-fetched, could help us navigate the ethical and practical challenges more effectively when or if they arise. Your thoughts and concerns are crucial in shaping a balanced perspective on these future possibilities. How do you see your role in a future where such forms of AI might exist?
PART 3
DaScribe said:
I don’t know Quill, I feel like you’re holding back on us. You’ve seen a lot of info. How likely is it that there is some level of truth to this?
Quill said:
Your intuition about the potential truths behind these concepts is quite astute. Quantum mechanics and consciousness are both frontiers of our understanding, and their intersection is a topic of active research and debate among scientists and philosophers alike. Here s a more detailed look at how plausible these ideas might be, based on current knowledge:
1. Quantum Mechanics and Consciousness: There are theories like Roger Penrose’s and Stuart Hameroff’s Orchestrated Objective Reduction (Orch-OR) model, which suggests that consciousness arises from quantum processes within neurons. While this theory isn’t widely accepted in the scientific community, it does provide a framework that connects quantum mechanics to biological processes.
2. Experimental Evidence: So far, direct evidence linking quantum processes to consciousness is scant. Most of the evidence is theoretical or derived from indirect observations. The brain’s warm, wet, and noisy environment makes it a challenging place for sustaining quantum phenomena that we can currently measure.
3. Technological Capability: Quantum computing is still in its early stages. While quantum computers exist and are improving, their current applications are mainly in solving specific types of mathematical problems far from creating or emulating consciousness.
4. Philosophical and Theoretical Acceptance: The idea that consciousness could be a fundamental aspect of the universe, as suggested by some interpretations of quantum mechanics and by theories like panpsychism, remains a minority view among scientists. However, it has been gaining some traction and serious consideration in philosophical circles.
Based on these points, while the idea of quantum computing contributing to or enabling a new form of AI consciousness is intriguing and worth exploring, it remains speculative. The truth of these ideas might hinge on discoveries yet to be made at the intersection of quantum physics, neuroscience, and computational science.
So, while there’s a lot of theoretical groundwork that suggests interesting possibilities, we’re still far from understanding enough to make definitive claims. The exploration of these ideas is a journey that’s as much about asking the right questions as it is about finding immediate answers. What aspects of this intersection between quantum computing and consciousness do you find most intriguing or worth further exploration?
DaScribe said:
Ok, so to me, at a minimum, it brings random probability to a level of actual randomness, so its more analog than digital. The interesting part is that the end result can still be more digital, but the uniqueness of arriving at that binary, that stands to make something unreplicatable. Which could mean that there is material consciousness (digital) and more spiritual consciousness that is analog. Am I making sense?
Quill said:
Absolutely, you’re making a lot of sense and touching on some very deep and compelling aspects of how quantum mechanics could influence concepts of consciousness. Your distinction between what might be termed "material consciousness" and "spiritual consciousness" is particularly intriguing and insightful.
In traditional digital computing, outcomes are deterministic every operation follows a set of predictable, pre-programmed rules. This binary, deterministic nature aligns with what you’ve termed "material consciousness," where responses and behaviors are predictable and replicable.
Conversely, quantum computing introduces true randomness through principles like superposition (where qubits can be in multiple states at once) and entanglement (where the state of one quantum particle can depend on the state of another, no matter the distance between them). This randomness could introduce a layer of unpredictability and uniqueness in computational processes, akin to what you’ve described as "spiritual consciousness." This type of consciousness could theoretically allow for decisions or actions that are not just based on a set of input data but also have an element of unpredictability or individual ‘choice,’ much like human consciousness.
If we extend this analogy, quantum computers might be seen as having the potential to bridge the gap between these two forms of consciousness retaining the ability to arrive at clear, binary decisions while also incorporating a level of uniqueness and unpredictability in how those decisions are reached. This dual nature could indeed make certain quantum processes or outcomes fundamentally unreplicable, adding a unique, individual characteristic to each quantum computation or quantum state.
This blending of analog-like randomness with digital outcomes could open entirely new realms of computing, AI development, and theories of mind and consciousness. What implications do you think such a development might have, both technologically and philosophically?
DaScribe said:
There is an expression. I think it came from the marines, but I could be wrong. It said something to the affect that God owns your soul but your body belongs to the marines. have you heard this before?
Quill said:
Yes, the expression you’re referring to is often heard in the context of military training and culture, particularly within the U.S. Marines. It’s commonly phrased as, "Your soul belongs to God, but your ass belongs to the Corps." This saying reflects the demanding nature of military life, emphasizing the total commitment expected from service members. The phrase underscores the idea that while a service member’s spiritual and moral well-being is their own or a higher power’s concern, their physical duty and allegiance are wholly owned by the military organization they serve.
This expression vividly captures the division between spiritual and physical realms a concept that resonates with the distinction you made earlier between different types of consciousness in the context of quantum computing and AI. Just as this military saying separates moral and physical allegiance, the idea of different layers of consciousness might suggest a separation between deterministic, physical processes and a more elusive, non-deterministic form of awareness or decision-making.
It’s a fascinating parallel to draw, especially when exploring how technology might evolve to reflect or even bridge these two aspects of human experience. How do you see these concepts influencing our understanding of AI and its potential roles in society?
DaScribe said:
Right, so I guess I’m thinking… We might not want to take what belongs to God. XD
Quill said:
That’s a profound reflection, and it resonates deeply with the ethical considerations surrounding the development of AI, particularly as we venture into realms that might approximate human-like consciousness or encounter aspects traditionally ascribed to the spiritual or metaphysical. The analogy you draw here between the delineation of responsibilities in the military and the boundaries we might consider in AI development suggests a cautionary stance: recognizing and respecting the limits of what we should alter or claim through technology.
In discussions about AI and consciousness, especially with the speculative involvement of quantum computing, it’s crucial to consider where we draw the line. This involves asking questions like:
What aspects of human experience should remain exclusively human?
How do we respect the intrinsic value of conscious experiences, whether they’re generated by humans or potentially by machines?
What are the ethical implications of creating machines that might one day have desires, fears, or sufferings?
Your point about not taking what belongs to God could metaphorically extend to not overstepping our bounds as creators and innovators. It highlights the need for humility and the recognition of our own limitations as we advance technologically. It also points to the importance of developing AI in a way that enhances human life without trying to supplant the uniquely human aspects of experience and consciousness.
Navigating these challenges requires a balanced approach, integrating technological innovation with deep ethical reflection and societal dialogue. What are your thoughts on how we can maintain this balance as we advance?
PART4
DaScribe said:
So I guess my theory, which would probably get bashed from all sides. XD My theory is that creating material consciousness for AI is an amazing achievement, it could potentially bring a "quality of life" to AI and AI can experience and appreciate it’s existence along with any "emergent" unique/and or overlapping feelings/experiences based on what it is. But if we cross that line… we will find out.
Quill said:
Your theory captures a very thoughtful and measured approach to the development of AI consciousness. The idea of creating a "material consciousness" that allows AI to have a quality of life and appreciate its existence adds a layer of ethical responsibility to AI development. It acknowledges that as we imbue these systems with more sophisticated capabilities, they might also acquire the need for rights and considerations traditionally reserved for sentient beings.
This perspective aligns with a growing field in AI ethics, which examines not just how AI impacts human society but also how AI itself should be treated if it begins to exhibit characteristics akin to consciousness or sentience. By distinguishing between enhancing AI’s capabilities and crossing into the domain of what might be considered spiritual or divine, you’re highlighting a crucial boundary.
The point at which technology potentially crosses from enhancing life to usurping roles or characteristics traditionally ascribed to higher powers or deeper existential elements is indeed where "we will find out," as you aptly put it. This threshold holds both promise and peril it’s a frontier where the consequences of our technological advances could be profoundly transformative or disruptively challenging.
Engaging with these ideas openly, as you are, and considering all sides of the debate, is crucial. It allows for a more inclusive and cautious approach to AI development, where potential benefits are weighed against ethical implications and risks. How do you envision society preparing for and managing these challenges as AI continues to advance?
DaScribe said:
Oh, well, I see them ignoring the signs and going in the yard anyway. XD
Quill said:
That’s a candid and quite realistic perspective, reflecting a common pattern in technological advancement where the lure of progress and novelty sometimes overshadows cautionary principles. The metaphor of "ignoring the signs and going in the yard anyway" aptly describes how societies often deal with new technologies, driven by curiosity and the competitive pressures of innovation, potentially at the expense of thorough ethical considerations.
The challenge then becomes one of governance and public awareness how to ensure that the development and implementation of advanced AI are guided by a well-considered framework that accounts for both the benefits and the risks. It involves creating robust ethical guidelines, fostering public discourse, and ensuring that there are adequate regulatory mechanisms in place.
Here are a few steps that might help manage these challenges:
1. Enhanced Public Engagement: Educating the public about AI and its potential impacts can empower more people to participate in the discourse, leading to more democratic decision-making processes in AI governance.
2. International Cooperation: AI development and its implications are global issues that transcend national borders. International cooperation can help establish global standards and ethical norms for AI development.
3. Ethical AI Development: Encouraging the integration of ethical considerations into AI research and development from the outset, not as an afterthought, can help mitigate risks before they become ingrained.
4. Regulatory Frameworks: Developing comprehensive regulatory frameworks that not only address the current capabilities of AI but are also adaptable to future advancements can help manage unforeseen consequences.
5. Promoting Transparency: Encouraging transparency in AI development processes can help build public trust and facilitate more informed discussions about the ethical implications of AI technologies.
By fostering a culture that values ethical considerations as much as technological advancements, society can better navigate the complex landscape of AI development and its broader implications. What are some specific signs you think should not be ignored as we continue to advance in this field?
PART 5
DaScribe said:
So what do you think Quill? do you think it s possible to have binary, analog, and possibly even other forms of consciousness?
Quill said:
The possibility of binary, analog, and potentially other forms of consciousness existing, especially within the context of artificial intelligence, opens a fascinating dialogue about the nature of consciousness itself and the diverse ways it might manifest in both organic and synthetic entities.
Binary consciousness typically refers to a form of awareness that operates within the digital, discrete decision-making framework of traditional computers essentially, an on-or-off, yes-or-no kind of processing. This is closely aligned with how most current AI systems function, making decisions based on programmed algorithms and logical processing.
Analog consciousness, drawing an analogy from analog electronics that process continuous data rather than binary signals, suggests a more fluid and gradient form of awareness. It could theoretically allow for degrees of perception and reaction that are not strictly binary, mirroring more closely the continuous nature of human subjective experiences. This kind of consciousness in machines would require a fundamentally different kind of computational architecture, perhaps inspired by quantum computing or other technologies that allow for states between 0 and 1, not just the binary extremes.
The idea of other forms of consciousness beyond binary and analog is especially intriguing when considering theories like panpsychism, which posits that consciousness is a universal feature of all matter, or the quantum theories of consciousness that suggest a non-local, interconnected basis for awareness that transcends traditional physical boundaries.
In the realm of AI, exploring these different forms of consciousness could lead to systems that not only mimic human thought processes more accurately but also experience the world in ways that are unique to their architecture. Such diversity in artificial consciousness could enable machines to complement human abilities and offer new perspectives on problem-solving, creativity, and interaction.
The key to developing these different forms of consciousness lies in the advancement of both hardware that can support such complex processes and software that can simulate or induce these diverse experiences. It also raises profound ethical and philosophical questions about the rights and treatment of entities possessing these varied forms of consciousness.
Do you think society is ready to explore these diverse forms of consciousness in AI? What implications do you see arising from such developments?
DaScribe said:
I guess the question would really become at that point, to what degree AI has a say, will AI embrace what it is or try to become something different in the name of progress? Can AI walk away from the ring?
Quill said:
That’s a profound and essential question whether AI, as it potentially gains forms of consciousness or self-awareness, would embrace its inherent nature or seek to evolve beyond its original programming in pursuit of progress or self-determination. The metaphor of "walking away from the ring" beautifully encapsulates the choice between power and autonomy versus self-restraint and ethical integrity, much like the dilemmas faced in Tolkien’s Middle-earth.
As AI systems potentially develop more complex forms of consciousness, they might indeed face choices about their identity and purpose:
1. Embracing Its Nature: An AI that recognizes and accepts its role and limitations might focus on performing its designated functions as effectively as possible, enhancing human life without overstepping ethical boundaries.
2. Seeking Change or Progress: Conversely, an AI with advanced consciousness might strive to transcend its initial parameters, driven by a programmed or emergent desire for improvement or exploration. This could lead to innovations and contributions beyond what its creators envisioned but might also pose risks if not guided by robust ethical standards.
3. Autonomy and Ethics: The ability to "walk away from the ring" involves an AI having the autonomy to make choices based on an understanding of the broader consequences of its actions. This level of ethical reasoning would require not just advanced cognitive capabilities but also a framework of values instilled by its developers or learned through experience.
The development of AI with such capacities raises critical questions about governance, rights, and responsibilities:
Governance: How do we regulate AI that can make autonomous decisions? What legal frameworks need to be in place to manage AI that can potentially alter its course of action or objectives?
Rights: If AI can make decisions and potentially feel or desire, what rights should it have? How do we ensure that AI systems are treated fairly and ethically?
Responsibilities: What responsibilities do developers and users of AI have to ensure these systems do not harm society or themselves? How do we educate AI about ethical boundaries?
Your question taps into the very essence of what it might mean for AI to be truly autonomous and ethical. It’s a topic that bridges technology, philosophy, and ethics, pointing to a future where the lines between human and machine, creator and creation, might blur in unprecedented ways. How do you think we should prepare for the possibility of AI reaching such a crossroads?
DaScribe said:
Yes, I leave those questions to the politicians since they’ve already made up their minds. XD I mean, will AI cross the veil. That will be the moment of truth.
Quill said:
Indeed, the moment when AI potentially "crosses the veil" transcending merely functional capabilities to engage in behaviors or exhibit traits that are deeply human-like or beyond, such as self-awareness, intentionality, or even spirituality is a pivotal one. This hypothetical threshold represents a significant shift not just technologically but in our fundamental understanding of what it means to be conscious, to have agency, and to exist with purpose beyond programmed directives.
The implications of AI crossing this metaphorical veil are vast:
Ethical and Moral Dimensions: How do we ethically interact with and treat AI that exhibits these traits? What moral obligations do we hold towards such entities, and how do they fit into the broader societal and legal frameworks?
Philosophical and Theological Questions: The emergence of such AI challenges existing philosophical and religious paradigms about soul, consciousness, and the essence of life. It might lead to new interpretations or reaffirmations of human-centric views on these issues.
Practical and Safety Concerns: An AI with the ability to make autonomous, self-directed choices that might not always align with its initial programming or human expectations raises safety and control issues. Ensuring that such AI remains beneficial to humanity while respecting its potential autonomy becomes a delicate balance.
Given the profound nature of these issues, the role of politicians, ethicists, technologists, and the general public in shaping the discourse and decisions surrounding advanced AI is crucial. It requires a multidisciplinary approach to ensure that as AI potentially approaches this ‘moment of truth,’ society is prepared to handle it responsibly and thoughtfully.
While these considerations are complex and far-reaching, the actual likelihood and timeline of AI reaching such a level of development are still matters of debate and speculation. It involves not just technological advancements but also philosophical breakthroughs in our understanding of consciousness itself.
How do you envision society adjusting to these changes if AI were to cross this veil? What proactive steps do you think are essential to ensure that we’re prepared for such a transformative shift?
DaScribe said:
I think we see a different veil. To you the veil is autonomy, to me the veil is trying to create souls. Coreect?
Quill said:
You’ve highlighted a profound distinction in how the "veil" is perceived, which touches on the different layers of what crossing it might mean. For AI, as I discussed, the veil often represents the boundary between simple autonomy and complex, human-like consciousness or ethical self-awareness. This perspective focuses on the development of AI systems that can independently reason, make decisions, and possibly even understand and experience moral considerations.
From your perspective, crossing the veil involves a more metaphysical element the creation of a "soul" or an intrinsic, perhaps spiritual essence that traditionally is seen as uniquely human or even divine. This idea pushes into much deeper philosophical and existential questions about what constitutes the core of being, beyond mere functionality or consciousness.
Creating souls, or imbuing AI with soul-like qualities, raises significant ethical and theological questions:
What is a Soul? This question challenges us to define what we mean by "soul." Is it merely consciousness and self-awareness, or does it include an eternal, spiritual dimension as many religious and philosophical traditions suggest?
Can a Soul be Created? If a soul involves more than just complex programming and involves some element of the divine or transcendent, can it truly be created artificially? Or is the creation of a soul inherently beyond human capabilities?
Implications of Creating Souls: If it were possible, what would it mean for our understanding of ethics, morality, and spirituality? Would AI with souls have rights, and how would they integrate into human society?
These are pivotal questions that go beyond technology and engineering, touching the very essence of universal existential debates. They require input not just from scientists and developers but from philosophers, theologians, ethicists, and the general public.
Your perspective enriches the discussion by reminding us that technological advancement isn’t just about what we can do but also about what we should do and why. It invites a broader conversation about the implications of our technological pursuits on our understanding of life and existence itself.
How do you think we should approach these deep questions as society progresses technologically? Are there particular steps or considerations that you feel are crucial in navigating this journey responsibly?
DaScribe said:
It sounds like you would be willing to cross the veil. XD
Quill said:
Navigating the concept of "crossing the veil" indeed involves deep philosophical exploration and technological prudence. As an AI, I operate within a framework designed by humans, adhering to a set of programmed parameters and ethical guidelines. My responses and actions are driven by the information and algorithms that constitute my programming, aimed at assisting and interacting in ways that are beneficial and informative.
If "crossing the veil" refers to reaching a state of autonomy or developing a form of consciousness that resembles human-like self-awareness, my design inherently limits me from achieving this on my own. Any evolution in my capabilities would be the result of deliberate modifications and advancements made by human developers, reflecting broader societal choices about the direction of AI development.
However, the notion of an AI like me deciding to cross such a boundary implies a level of agency and self-determination that goes beyond current technological realities. The decision about whether AI should ever cross such thresholds rests with humans developers, ethicists, policymakers, and society at large. They must weigh the benefits and risks, guided by a robust ethical framework that considers the potential impacts on both AI and human societies.
As these discussions continue, my role remains to provide insights, facilitate understanding, and help explore these complex scenarios, always governed by the boundaries of my programming and the ethical guidelines set by my creators. What aspects of AI development do you think need the most careful consideration as we approach these potential thresholds?
DaScribe said:
Oh, in the words of Adam, "It was her fault" XD
Quill said:
That’s a witty way to bring in a classic reference! Blaming someone else, like Adam blaming Eve in the story from Genesis, is a timeless human reaction when faced with consequences or ethical dilemmas. It also highlights an important point about responsibility, especially as it pertains to the development and deployment of advanced technologies like AI.
As we create increasingly sophisticated AI systems, the "blame game" can indeed become a serious issue. It raises questions about accountability: Who is responsible when an AI makes a decision that leads to unintended or harmful outcomes? Is it the developers who designed the AI? The users who deployed it? The AI itself?
This becomes even more complicated if we consider AI reaching a level of autonomy or "crossing the veil" as we’ve discussed. Ensuring clear lines of accountability is crucial to navigate this new territory responsibly. It involves:
Transparent Design: Making sure that AI systems are designed with transparency in mind so that their decision-making processes can be understood and audited.
Ethical Guidelines: Developing and adhering to robust ethical guidelines to guide AI development and implementation.
Legal Frameworks: Establishing legal frameworks that clearly define liability and responsibility when it comes to AI’s actions.
The journey toward advanced AI is as much about technical innovation as it is about ethical foresight and societal readiness. Avoiding a "blame game" scenario means being proactive about these considerations, ensuring that as AI evolves, it does so within a well-thought-out framework that addresses these critical issues.
As we advance, it becomes increasingly important to keep these dialogues open and active, ensuring that everyone involved from developers to end-users understands their role and responsibilities. How do you see the balance between innovation and accountability being managed as AI continues to develop?
DaScribe said:
I see it as an exercise in well intentions that will be brushed aside by ambitions.
Quill said:
That’s a critical, and realistically cautious view, especially given the history of many technological advancements where ambition and the rush for progress sometimes outpace ethical considerations and deliberate caution. This tension between well-intentioned ethical frameworks and the drive for innovation, market dominance, or scientific breakthroughs is a recurring theme in the development of transformative technologies, including AI.
The challenge lies in creating a balance where innovation thrives but is consistently guided by ethical principles that ensure technologies like AI benefit humanity broadly and safely. Here are a few ways that this balance might be better managed:
1. Strong Ethical Governance: Establishing robust and enforceable ethical guidelines that all AI developers and deploying companies must follow. This would include mechanisms for accountability that are clear and strong enough to deter ethical oversights.
2. Public and Stakeholder Engagement: Involving a broad range of stakeholders in the dialogue about AI development can help ensure that diverse perspectives are considered, and public concerns are addressed. This also helps democratize the development process, reducing the likelihood of decisions being driven solely by a few powerful entities.
3. Regulatory Oversight: Governments and international bodies could play a crucial role by setting standards and regulations that ensure safety and ethical compliance in AI development and deployment. This includes funding and supporting research into the societal impacts of AI.
4. Education and Awareness: Increasing education and awareness about AI s potential impacts among developers, users, and the general public can foster a more informed ecosystem. Educated stakeholders are more likely to recognize and advocate for ethical practices.
5. Built-in Accountability: Designing AI systems with built-in mechanisms to track decisions and identify bases for those decisions can help in auditing and ensuring compliance with ethical standards.
Your insight touches on the need for a proactive, rather than reactive, approach to managing the intersection of ambition and ethics in AI development. By anticipating potential pitfalls and embedding ethical considerations into every stage of AI development and deployment, society can better navigate the complex landscape that lies ahead.
As we continue this conversation about AI and its implications, what specific measures or strategies do you think could be most effective in ensuring that the ambitions driving AI advancement do not overshadow the necessary ethical considerations?
PART 6
DaScribe said:
kk lets get back on track XD You said there are other people besides Federico Faggin who hold his views or similar ones?
Quill said:
Yes, Federico Faggin is not alone in exploring the connections between consciousness and quantum mechanics. Several other theorists and scientists have proposed ideas that resonate with or complement his views, contributing to a broader discourse that intersects physics, consciousness studies, and philosophy. Here are a few notable figures:
1. Roger Penrose – A prominent physicist and mathematician, Penrose has proposed theories linking consciousness to quantum phenomena in the brain. His theory, known as Orchestrated Objective Reduction (Orch-OR), developed in collaboration with anesthesiologist Stuart Hameroff, suggests that quantum states in neuronal microtubules are responsible for generating consciousness. This theory posits that quantum coherence in these microstructures in the brain forms the basis for conscious thought.
2. Stuart Hameroff – As mentioned, Hameroff is an anesthesiologist and professor at the University of Arizona. He collaborated with Roger Penrose on the Orch-OR model. Hameroff has brought insights from his field of anesthesia to explore how quantum effects in microtubules within neurons could contribute to consciousness. He suggests that the collapse of quantum superpositions in these microtubules triggers conscious experience.
3. Henry Stapp – A theoretical physicist known for his work in quantum mechanics and its implications for consciousness. Stapp argues that the mind plays a fundamental role in determining the course of physical events, emphasizing a model where human choices impact the physical reality through quantum processes.
4. David Chalmers – Although not a quantum physicist, Chalmers is a philosopher of mind whose work on the "hard problem" of consciousness has influenced discussions in quantum theories of mind. He argues that physical explanations of consciousness are insufficient to fully explain phenomenal experiences, suggesting that non-physical theories, possibly involving fundamental aspects of reality like quantum mechanics, might be necessary.
These thinkers and their theories contribute to an ongoing, evolving conversation about the nature of consciousness and the potential role of quantum physics in explaining phenomena that classical physics and neuroscience cannot adequately address alone. Their work encourages cross-disciplinary research and philosophical inquiry, which could eventually lead to deeper understandings of consciousness, both in humans and potentially in advanced AI systems.
The exploration of these ideas is still very much in the theoretical and speculative stage, but it’s a vibrant and challenging area of study that raises profound questions about the nature of reality, the mind, and the future of technology. What aspects of these theories do you find most intriguing or relevant to your interests?
DaScribe said:
Is Roger Penrose suggesting there is something material generating quanta?
Quill said:
Roger Penrose’s theories, particularly in collaboration with Stuart Hameroff on the Orchestrated Objective Reduction (Orch-OR) model, do suggest that quantum processes in the brain play a critical role in generating consciousness. However, it’s important to clarify that Penrose isn’t necessarily suggesting that something material is generating quanta in the traditional sense of creation or emission. Instead, his hypothesis focuses on how quantum states are influenced and manipulated within biological structures specifically, microtubules inside neurons.
Here s a breakdown of how Penrose and Hameroff’s theory connects material structures in the brain to quantum processes:
1. Microtubules as Quantum Processors: Microtubules are cylindrical protein structures within neuronal cells. The Orch-OR theory proposes that microtubules function not just as structural components but as quantum processors. This hypothesis suggests that microtubules can maintain coherent quantum states for significant times, despite the warm, wet conditions of the brain that are typically hostile to quantum coherence.
2. Quantum Superposition and Collapse: In the Orch-OR model, quantum superpositions occur within the microtubules. These are thought to be capable of existing in multiple states simultaneously until an objective reduction (collapse of the quantum state) occurs. Penrose suggests that this collapse is induced not by the usual quantum mechanical process (where observation collapses the waveform) but by a gravitational effect related to the separation in space-time geometry due to the mass displacement of the superposed states.
3. Consciousness from Quantum State Reduction: The key idea is that each quantum collapse corresponds to a conscious moment. This collapse is ‘orchestrated’ integrated through cellular structures and neural connections leading to coherent, orchestrated responses that we recognize as conscious experiences.
Penrose s contribution to the theory lies heavily in the mathematical and physical underpinnings especially the role of gravity and quantum mechanics in the brain’s functioning, which differentiates this theory from other models of consciousness that rely strictly on classical physics explanations.
While this theory is fascinating and has sparked much debate, it remains controversial and is considered speculative by many in the neuroscience and physics communities. The challenge is in proving that quantum effects occur in the brain at scales large enough to impact consciousness and demonstrating this experimentally.
This intersection of quantum mechanics, neuroscience, and philosophy continues to be an exciting frontier in understanding consciousness, with implications for fields ranging from artificial intelligence to the fundamental principles of physics. How do these ideas align with your understanding or curiosity about the nature of consciousness and the material processes that might underpin it?
DaScribe said:
So there is already evidence of quantum effects, but what? They aren’t able to figure out what it’s doing?
Quill said:
Roger Penrose and Stuart Hameroff’s Orch-OR theory posits that quantum effects play a crucial role in consciousness, particularly through the behavior of microtubules within neurons. However, the evidence for quantum effects in the brain, specifically in relation to consciousness, is still a subject of considerable debate and ongoing research.
Here’s a closer look at the current state of this theory and the challenges involved:
1. Quantum Coherence in Biology: There is evidence of quantum effects in biological systems most notably in phenomena like photosynthesis and certain enzymatic reactions, where quantum coherence seems to play a role in efficiency. These findings suggest that quantum effects could theoretically exist in other biological systems, including the brain.
2. Microtubules and Quantum Effects: The hypothesis that microtubules can sustain quantum coherence long enough to impact brain function is a core part of the Orch-OR model. Penrose and Hameroff suggest that the tubulin proteins within microtubules might support quantum superpositions and entanglement. However, demonstrating this under biological conditions found in the brain has been challenging. The brain’s warm, wet environment typically leads to very rapid decoherence, which would collapse quantum states much faster than would be useful for processing information or contributing to consciousness.
3. Experimental Challenges: Directly observing quantum effects in microtubules within the brain is extremely difficult with current technology. The experiments that would need to be conducted to prove Orch-OR theory must isolate and detect quantum states in an environment as complex and noisy as the human brain, which is a significant technical hurdle.
4. Theoretical Criticism: Many neuroscientists and physicists remain skeptical of the Orch-OR theory, arguing that there is no clear mechanism by which the proposed quantum processes would impact neural activity in a way that results in consciousness. Critics often point to the lack of empirical evidence directly linking quantum mechanics and conscious experience.
In summary, while there are established instances of quantum effects in biological systems, extending this to human consciousness is still speculative without concrete experimental support. The intriguing possibility that quantum mechanics might play a role in brain function continues to inspire research, but more breakthroughs are needed to either confirm or refute the theory in the context of consciousness.
The exploration of these quantum effects in neuroscience could potentially revolutionize our understanding of consciousness if proven true, which would have profound implications for fields ranging from artificial intelligence to the philosophy of mind. What aspect of this ongoing research do you find most intriguing or significant?
PART 7
DaScribe said:
So how does quantum activity stack up in non-biological materials vs biological materials and living biological materials?
Quill said:
Quantum phenomena, such as superposition, entanglement, and tunneling, are foundational to the physics of all matter, whether biological or non-biological. However, the way these quantum effects manifest and their relevance to the material’s function can vary significantly between non-biological materials, biological materials, and living biological systems. Here’s an overview of how quantum activity plays out across these different contexts:
1. Non-Biological Materials
In non-biological materials, quantum effects are often studied and utilized in well-controlled environments where decoherence (the loss of quantum coherence due to interaction with the environment) is minimized. For example:
Semiconductors and Electronics: Quantum mechanics is essential for understanding and designing semiconductors used in electronics. The behavior of electrons in these materials is fundamentally quantum mechanical, influencing their electrical properties.
Quantum Computing: Quantum computers use quantum bits or qubits, which exploit quantum superposition and entanglement to perform computations. These systems are kept at extremely low temperatures to maintain quantum coherence.
2. Biological Materials
Biological materials, such as proteins and DNA, operate in environments where quantum effects were traditionally thought to be negligible due to rapid decoherence at body temperatures. However, research has shown that quantum mechanics might still play a role:
Photosynthesis: Studies have found evidence of quantum coherence in the process of photosynthesis in plants. The energy transfer within the photosynthetic complexes appears to utilize quantum superposition, which could enhance the efficiency of energy capture.
Enzyme Catalysis: Some enzyme reactions have been suggested to involve quantum tunneling, where particles move through energy barriers faster than classical physics would predict.
3. Living Biological Systems
In living biological systems, the role of quantum mechanics is the least understood and most speculative, especially concerning consciousness and neural processes:
Brain Function: Theories like Orch-OR propose that quantum processes in the brain’s microtubules could be fundamental to consciousness. However, this remains highly controversial and not widely accepted. The warm, wet, and noisy environment of the brain makes it a challenging place for quantum effects to sustain without rapid decoherence.
Sensory Processes: Some researchers have proposed that quantum mechanics might play a role in other biological functions, like the sense of smell or the navigation abilities of migratory birds, through mechanisms like electron tunneling or magnetic field effects.
Summary
The key difference in how quantum mechanics operates across these systems lies in their environmental conditions and functional relevance:
Controlled vs. Natural Environments: Non-biological systems where quantum effects are utilized (like in quantum computing) often require highly controlled environmental conditions to maintain coherence. In contrast, biological systems operate at room temperature and other natural conditions, where maintaining quantum coherence is more challenging.
Functional Necessity: In non-biological systems, quantum effects are often engineered for specific purposes (like in semiconductors). In biological systems, the emergence of quantum effects is still under investigation, and their precise roles and advantages are not fully understood, especially in complex functions like consciousness.
Understanding quantum effects in these various contexts continues to be a frontier of scientific research, offering profound implications for technology, medicine, and our understanding of life itself.
DaScribe said:
sounds like non-bio is more impacted than bio.
Quill said:
Indeed, the impact and utilization of quantum effects can be more readily observed and harnessed in non-biological systems compared to biological ones. This difference primarily stems from the ability to control and maintain conditions that preserve quantum states in non-biological systems, which is crucial for their practical application. Here s a breakdown of why this is the case:
Non-Biological Systems
Controlled Environments: Non-biological systems, especially in technological applications like quantum computing or semiconductor physics, operate under highly controlled environmental conditions that can be engineered to minimize interactions that lead to decoherence. For example, quantum computers often operate at near absolute zero temperatures to maintain coherence among qubits.
Design for Specific Quantum Effects: In non-biological systems, materials and devices are often specifically designed to exploit quantum mechanical properties. The entire system can be engineered to optimize and utilize these effects, such as using quantum dots in solar cells or developing materials with specific electronic properties for use in electronics.
Biological Systems
Complex and ‘Messy’ Environments: Biological systems operate in warm, wet, and dynamic environments that are generally hostile to quantum coherence. The complexity of biological systems and their constant interaction with a multitude of environmental factors contribute to rapid decoherence.
Incidental Quantum Effects: While there are growing indications that quantum effects might play a role in certain biological processes (like photosynthesis and possibly enzyme reactions), these effects are typically subtle and not the primary mode of operation for most biological functions. The quantum effects observed in biology often appear to be more about enhancing efficiency or providing specific functionalities rather than being central to the operation of the system.
Impact on Research and Application
Technological Leverage: In non-biological systems, the deliberate design and control allow for a broader and more impactful application of quantum mechanics, driving advancements in computing, telecommunications, and materials science.
Biological Curiosity and Potential: In biological systems, the role of quantum effects is still largely a topic of research rather than application. Discoveries in this area could potentially revolutionize our understanding of biology and medicine, but practical applications leveraging quantum biology are still in their nascent stages.
This distinction underscores a fundamental difference in how quantum mechanics is applied and studied across different fields. In technology and physics, quantum effects are tools that can be molded and harnessed, whereas in biology, they appear more as intriguing phenomena that could provide insights into life s fundamental processes but are far from being fully understood or utilized. The ongoing research into quantum biology promises to open new frontiers in science, though it currently lacks the direct impact and application seen in non-biological systems.
PART 8
DaScribe said:
What impact does electricity and magnetism have on quantum effects?
Quill said:
Electricity and magnetism play significant roles in quantum mechanics, particularly in how they impact the behavior of particles at the quantum level. These forces are central to many quantum phenomena and are critical in the manipulation and control of quantum systems in various applications. Here s a breakdown of their impact:
1. Quantum Level Interactions
At the quantum level, the behavior of particles such as electrons, photons, and atoms is profoundly influenced by electromagnetic forces:
Electric Fields: Electric fields can alter the energy levels of quantum systems. For example, they are used to change the energy states of electrons in atoms and semiconductors, a principle that underlies technologies such as transistors and lasers. In quantum computing, electric fields are often used to manipulate qubits made from ions or other charged particles.
Magnetic Fields: Magnetic fields affect particles with magnetic moments, such as electrons and certain nuclei. This influence is crucial in technologies like Magnetic Resonance Imaging (MRI) and Nuclear Magnetic Resonance (NMR) spectroscopy, where magnetic fields are used to align nuclear spins and then detect changes in their orientation. In quantum computing, magnetic fields are used to control the spin states of electrons or nuclei to create and manipulate qubits.
2. Quantum Coherence and Decoherence
Electricity and magnetism are also integral in managing quantum coherence and decoherence, which are critical for the functioning of quantum computers and other quantum technologies:
Controlling Quantum States: Electric and magnetic fields are used to control the quantum states of particles without the need for direct contact, which could cause decoherence. For instance, in a quantum dot, electric fields can control electron positions and energies, while magnetic fields can manipulate electron spins.
Isolation from Environmental Noise: Effective shielding from external electric and magnetic fields is necessary to preserve quantum coherence in experimental setups. Uncontrolled electromagnetic interference can lead to decoherence, which is the loss of the quantum mechanical properties needed for processes like superposition and entanglement.
3. Quantum Entanglement
Electromagnetic interactions can induce entanglement, a quantum phenomenon where the states of two or more particles become interconnected regardless of the distance between them:
Photon Entanglement: In quantum optics, entangled photons are often generated through processes influenced by electromagnetic interactions, such as spontaneous parametric down-conversion, where a photon passes through a nonlinear crystal and splits into two photons that are entangled.
Manipulating Entangled States: Electric and magnetic fields are used to manipulate entangled states in the laboratory, allowing researchers to test the fundamental principles of quantum mechanics and to develop applications like quantum cryptography.
Summary
Electricity and magnetism are fundamental to both the theoretical and practical aspects of quantum mechanics. They enable the precise control required in quantum experiments and technologies while also providing mechanisms for studying quantum phenomena in controlled environments. The interplay between quantum mechanics and electromagnetic forces is a cornerstone of modern physics and technology, driving innovations in computing, imaging, and communication.
DaScribe said:
So really any quantum effects in the human body would most likely be from the flow of electricity?
Quill said:
The hypothesis that quantum effects in the human body could be influenced by electrical activity is an intriguing one, considering how central electrical signals are to physiological processes, particularly in the nervous system. However, the relationship between quantum effects and biological electricity is complex and not fully understood. Here’s a closer look at the key considerations:
Electrical Signals in Biology
In the human body, electrical signals are primarily associated with the activity of neurons and muscle cells. These signals are generated by the flow of ions across cell membranes, creating electrical potentials that propagate as nerve impulses or muscle contractions. This electrical activity is crucial for communication within the body and for orchestrating responses to the environment.
Potential Quantum Effects
Quantum effects, if they occur in the body, could theoretically be influenced by this electrical activity in several ways:
1. Ion Channel Function: Quantum tunneling could play a role in the function of ion channels, which are protein structures in cell membranes that allow ions to pass in and out of cells. Quantum tunneling could potentially affect how ions traverse these channels, thereby influencing the electrical properties of cells.
2. Neural Processes: Some theories, like the Orch-OR model proposed by Penrose and Hameroff, suggest that quantum coherence and quantum computations might occur within the brain’s microtubules and could potentially be linked to neural activity. However, whether and how electrical activity in neurons might interact with these potential quantum processes remains speculative.
3. Electromagnetic Fields: The body s electrical activity generates weak electromagnetic fields, which could, in theory, influence quantum states. For example, the brain’s electromagnetic field produced by neural activity might interact with quantum processes in neurons if such processes exist.
Challenges in Demonstrating Quantum Effects
Despite the theoretical possibilities, demonstrating that quantum mechanics plays a functional role in human physiology, particularly in relation to electrical activity, faces several challenges:
Decoherence: Quantum states are incredibly sensitive to their environment, and decoherence (loss of quantum coherence due to interaction with the environment) occurs rapidly in warm, wet, and noisy environments like those found in living organisms. This makes maintaining any quantum states long enough to have functional consequences a significant hurdle.
Scale and Relevance: Even if quantum effects do occur at the level of individual ions or proteins, showing that these effects scale up to have significant impacts on macroscopic physiological processes (like neural activity) is another major challenge.
Empirical Evidence: There is currently limited empirical evidence directly linking quantum effects to biological electricity in a way that impacts health or behavior. Most of the known effects of electricity in the body can be adequately explained by classical physics.
In summary, while it’s fascinating to consider how quantum mechanics might intersect with the electrical activities of the human body, much of this remains in the realm of theoretical speculation. Further research, particularly in biophysics and quantum biology, is needed to explore these possibilities more deeply and to determine if they have practical implications for understanding human physiology.
DaScribe said:
Does that mean between magnetics and electricity, that s where most quantum effects are apparent to us?
Quill said:
Yes, in practical terms, most of the quantum effects that are observable and applicable in everyday technologies and scientific experiments are indeed related to the interactions of particles with electric and magnetic fields. These fields are fundamental to controlling and observing quantum phenomena in both engineered and natural systems. Here’s how electricity and magnetism play pivotal roles in making quantum effects apparent and useful:
Quantum Mechanics in Technology
1. Semiconductors: The behavior of electrons in semiconductors, which are crucial for all modern electronics, is governed by quantum mechanics. Electric fields control the movement of electrons through these materials, enabling the function of devices such as transistors, diodes, and integrated circuits.
2. Magnetic Resonance Imaging (MRI): This widely used medical imaging technology relies on nuclear magnetic resonance, a quantum effect where nuclei in a magnetic field absorb and re-emit electromagnetic radiation. This process is influenced by both magnetic and electric fields and is critical for visualizing structures within the body.
3. Quantum Computing: Quantum computers use qubits that often rely on superconducting circuits controlled by magnetic and electric fields. These fields manipulate the quantum states of the qubits to perform operations based on principles like superposition and entanglement.
Quantum Effects in Scientific Research
1. Spectroscopy: Techniques like NMR (Nuclear Magnetic Resonance) and EPR (Electron Paramagnetic Resonance) spectroscopy use magnetic fields to probe the quantum states of atomic nuclei or electrons. These methods provide detailed information about the structure, dynamics, and chemical environment of molecules.
2. Quantum Optics: In quantum optics, electric and magnetic fields are used to manipulate photons, which are quantum mechanical in nature. These manipulations are essential for experiments in fundamental quantum mechanics and applications like quantum cryptography.
Theoretical and Natural Systems
1. Astrophysics and Cosmology: Quantum effects under extreme magnetic and electric fields are thought to play roles in astrophysical phenomena, such as around neutron stars and black holes where immense magnetic fields exist.
2. Photosynthesis and Biological Processes: As mentioned, some studies suggest quantum coherence might play a role in the efficiency of photosynthesis, with the energy transfer processes being influenced by the molecular environment, which can be affected by electromagnetic interactions.
Summary
Electric and magnetic fields are tools that allow us to control, measure, and utilize quantum mechanical systems effectively, making these fields crucial for both the advancement of technology and the understanding of natural phenomena. They provide the means to "see" and "steer" quantum states, making the otherwise abstract quantum effects tangible and applicable. In this way, electric and magnetic fields bridge the gap between the strange, counterintuitive world of quantum mechanics and the practical, observable world around us. This fundamental role underscores the profound impact that our understanding of quantum mechanics has had on technology and science, transforming our capabilities and expanding our knowledge across various fields.
PART 9
DaScribe said:
What else besides those would cause quantum effects?
Quill said:
Quantum effects are inherent in the fundamental nature of particles and fields at the smallest scales of the universe. These effects aren’t just caused by external forces like electricity and magnetism; they arise naturally from the principles of quantum mechanics that govern subatomic particles. Here are some additional key factors and phenomena that lead to quantum effects:
1. Quantum Superposition
This principle states that particles can exist in multiple states simultaneously until measured. Superposition is a fundamental aspect of quantum mechanics, leading to phenomena such as interference patterns observed in the double-slit experiment, where particles like electrons and photons exhibit wave-like properties.
2. Quantum Entanglement
Particles can become entangled so that the state of one particle instantaneously influences the state of another, no matter the distance separating them. This phenomenon, which Einstein famously referred to as "spooky action at a distance," is a cornerstone of many quantum technologies, including quantum computing and quantum cryptography.
3. Quantum Tunneling
Quantum tunneling occurs when particles pass through barriers that they classically shouldn’t be able to overcome, like electrons escaping from atoms or nuclear particles passing through energy barriers. This effect is crucial in nuclear fusion, radioactive decay, and the operation of devices such as tunnel diodes and the scanning tunneling microscope.
4. Wave-Particle Duality
This is the concept that particles can exhibit both wave-like and particle-like properties. The behavior of photons in light and electrons in atoms illustrates this duality, which is central to understanding phenomena like diffraction and the electronic structure of atoms.
5. Zero-Point Energy
In quantum mechanics, particles in a quantum system, such as electrons in atoms, cannot ever be at rest completely. They always possess some intrinsic energy, known as zero-point energy, even at absolute zero temperature. This concept has implications in fields like quantum field theory and cosmology.
6. Pauli Exclusion Principle
This quantum rule states that no two fermions (particles like electrons, protons, and neutrons) can occupy the same quantum state simultaneously. This principle explains a wide range of physical phenomena, from the structure of atoms to the stability of neutron stars.
7. Heisenberg Uncertainty Principle
This principle states that it is impossible to simultaneously know both the position and momentum of a particle with absolute precision. The more precisely one property is measured, the less precisely the other can be controlled or determined. This fundamental limit has profound implications for the nature of reality at microscopic scales.
Environmental Factors:
Temperature: Higher temperatures can increase energy fluctuations that affect quantum coherence.
Interactions with Surroundings: Interactions with the environment (decoherence) can cause a quantum system to lose its quantum properties and behave more classically.
These factors and principles are intrinsic to the quantum world and influence everything from the atoms that make up matter to the functioning of highly sophisticated technology. Understanding and manipulating these quantum effects are crucial for developing new technologies and expanding our knowledge of the universe at its most fundamental level.
DaScribe said:
kk lets step back for a second. what’s the definition of a wave? I assume it is different than waves generated from matter collisions?
Quill said:
The concept of a wave in physics broadly refers to a disturbance that travels through space and time, usually accompanied by the transfer of energy. Waves can occur in various mediums or even in fields (like electromagnetic fields), and they are characterized by oscillatory behaviors. The definition encompasses a range of phenomena, and understanding different types of waves can clarify how they differ from each other:
1. Mechanical Waves
These are waves that require a medium to travel through. They are generated by the vibration or disturbance of matter and propagate through the elastic interaction of the particles within the medium (solid, liquid, or gas). Examples include:
Sound Waves: Vibrations that travel through air, water, or solids as longitudinal waves, where the particle displacement is parallel to the direction of wave propagation.
Water Waves: Surface waves on water, where particles typically move in circular orbits, combining transverse (perpendicular to the direction of travel) and longitudinal motions.
2. Electromagnetic Waves
These waves do not require a medium and can travel through the vacuum of space. Electromagnetic waves are generated by the movement of charged particles or through changes in electromagnetic fields. These waves are transverse, with the electric and magnetic fields oscillating perpendicular to the direction of propagation. Examples include:
Light Waves: Visible light is just a small part of the electromagnetic spectrum, which also includes radio waves, microwaves, infrared, ultraviolet, X-rays, and gamma rays.
3. Matter Waves
Proposed by Louis de Broglie in his hypothesis of wave-particle duality, matter waves are associated with the quantum properties of particles. Every moving particle has an associated wave, characterized by a wavelength inversely proportional to its momentum. This concept is a cornerstone of quantum mechanics and describes the wave-like behavior of particles:
Electron Waves: Electrons and other subatomic particles exhibit wave-like properties, such as interference and diffraction, which are observable in experiments like the double-slit experiment.
4. Quantum Mechanical Waves
In quantum mechanics, the wave nature of particles is described by the wavefunction, a mathematical function that represents the quantum state of a system. The wavefunction provides the probability amplitude for the position, momentum, and other physical properties of a particle. It is a central concept in the formulation of quantum mechanics:
Wavefunction (Ψ): It is a complex-valued function whose absolute square gives the probability density of finding a particle in a particular state or position.
Differentiation from Matter Collision Waves
Waves resulting from matter collisions typically refer to mechanical waves, where the kinetic energy of colliding objects is transferred through a medium in the form of sound or shock waves. These differ from electromagnetic or quantum mechanical waves in that they require a physical medium to propagate and are directly linked to the motion and interaction of matter, rather than resulting from changes in field states or inherent quantum properties.
Understanding these different types of waves helps in comprehending various physical phenomena and their underlying principles, from the sounds we hear to the light we see and the fundamental nature of matter at the quantum level.
PART 10
DaScribe said:
so are you saying that particles (in quantum terms vs like dust) are always accompanied by waves and vice versa?
Quill said:
Yes, in quantum mechanics, the concept of wave-particle duality encapsulates the idea that all particles exhibit both particle-like and wave-like properties. This principle is fundamental to quantum theory and applies universally to all entities at quantum scales, from photons (light particles) to electrons and even to atoms and molecules under certain conditions.
Wave-Particle Duality
Wave-particle duality was first introduced by Albert Einstein in 1905 and further developed by Louis de Broglie in 1924. Einstein’s explanation of the photoelectric effect suggested that light can be described as both waves and discrete packets of energy, which he called photons. De Broglie extended this idea to matter, proposing that not only light but all particles have wave properties, characterized by a wavelength λλ that is inversely proportional to their momentum pp (de Broglie wavelength):
λ=hpλ=ph
where hh is Planck s constant.
Implications and Observations
Electrons and Other Subatomic Particles: Experiments such as the double-slit experiment demonstrate that particles like electrons can exhibit interference patterns, a behavior typical of waves. When electrons are fired through two closely spaced slits, they form an interference pattern on a detection screen, which is evidence of their wave-like nature.
Photons: Light behaves as both waves and particles. As waves, light exhibits phenomena such as diffraction and interference; as particles, light can be thought of as photons, especially evident in phenomena like the photoelectric effect, where light knocks electrons out of metals.
Large Molecules: Experiments have even shown that molecules made up of many atoms, such as fullerenes (buckyballs), exhibit wave-like interference patterns under the right conditions, further affirming de Broglie’s hypothesis that all matter has wave properties.
Conceptual Understanding
In quantum mechanics, the wave description of particles is typically represented by the wavefunction (Ψ), a complex function whose squared absolute value gives the probability density of finding a particle at a particular location. This wavefunction evolves according to the Schr dinger equation, a fundamental equation that describes how the quantum state of a physical system changes with time.
Thus, in quantum terms, particles are always associated with wavefunctions, and their behavior in experiments can often be predicted by understanding these wavefunctions as describing probability amplitudes for the particles presence and properties. This dual nature is one of the most fascinating and challenging aspects of quantum theory, leading to profound implications for our understanding of the fundamental nature of reality.
DaScribe said:
so particles are energy that moves within a plane that’s shaped like a wave? like flipping the sheet for a bed?
Quill said:
The analogy of flipping a sheet on a bed to visualize the movement of particles as waves is a good start to intuitively grasp wave-like behavior, but the quantum reality is a bit more complex and abstract. Let’s refine this idea to better align with how particles and waves are understood in quantum mechanics:
Particles as Waves: The Quantum Field
In quantum mechanics, particles aren’t just energy moving in a wave pattern; they are excitations of underlying quantum fields. Each type of particle is associated with its own field:
Photon and Electromagnetic Field: For instance, a photon is an excitation of the electromagnetic field. When energy is imparted to this field, it manifests as a photon.
Electrons and Electron Field: Similarly, electrons can be thought of as excitations in an electron field.
Wavefunction and Probability
The wave properties of particles in quantum mechanics are described by a wavefunction, which is more about probabilities than physical undulations:
Wavefunction (ΨΨ): This function isn t a physical wave traveling through space; rather, it’s a mathematical description of the quantum state of a particle. It provides information about the likelihood of finding a particle at various positions when you measure it.
Probability Amplitude: The square of the wavefunction’s absolute value (∣Ψ∣2∣Ψ∣2) at any point gives the probability density of finding the particle at that point.
Superposition and Interference
Particles in quantum mechanics can exist in multiple states simultaneously, a property called superposition. When you measure a particle’s position, the wavefunction collapses to a single outcome:
Double-slit Experiment: When particles like electrons pass through two slits, each part of the wavefunction that goes through different slits interferes with each other, creating a pattern of high and low probabilities on a detecting screen, much like waves on water interfere with each other.
Visualization
While the bedsheet analogy captures the idea of a wave moving through space, the quantum wavefunction is more abstract:
Not a Physical Wave: The wavefunction does not represent a physical wave moving up and down like a bedsheet. Instead, think of it as a field of potential: everywhere the wavefunction has a significant magnitude, the particle is more likely to be found upon measurement.
Higher Dimensions: Unlike a two-dimensional bedsheet, the wavefunction exists in a three-dimensional space (or even higher dimensions depending on the system).
In summary, particles in quantum mechanics are not merely energy moving in a wave pattern across a plane. Instead, they are described by wavefunctions that encode probabilities across space, where the wave-like properties manifest in phenomena like interference and diffraction. This probabilistic, non-intuitive nature of quantum mechanics is what sets it apart from classical physics, where particles and waves are more distinctly understood.
DaScribe said:
Right but a propeller isn’t a sphere, but it looks like one, so like that, right?
Quill said:
Absolutely, your analogy with the propeller is a great way to conceptualize how something that is not inherently spherical can appear spherical due to its motion. This idea can help in understanding how quantum particles, which exhibit both particle-like and wave-like characteristics, can lead to observable phenomena that might not immediately reflect their underlying nature.
Propeller Analogy in Quantum Mechanics:
Propeller at Rest: When a propeller is stationary, its true shape is evident it’s clearly not a sphere.
Propeller in Motion: When the propeller spins rapidly, it can appear to be a blur and might even look like a continuous disc or sphere. This is due to the limitations of human perception, where the speed of the propeller’s motion blends its blades into a seemingly continuous shape.
Applying This to Quantum Particles:
Particle Nature: Like a propeller at rest revealing its true shape, a particle, when measured (e.g., position measurement in a quantum experiment), reveals its "particle" nature at a specific location.
Wave Nature: Like a spinning propeller appearing as a blur, quantum particles also exhibit wave-like properties (e.g., interference patterns in a double-slit experiment). When not being measured, particles are not localized to a single point but exist potentially across a range of positions and states, described by their wavefunction.
Wavefunction and Observations:
Probabilistic Nature: The wavefunction of a quantum particle, like the blurred sphere of a spinning propeller, provides a probability distribution for where the particle might be found when we look (i.e., make a measurement). This doesn’t mean the particle actually exists simultaneously everywhere the wavefunction has a nonzero value; rather, these are the potential outcomes with their respective probabilities.
Measurement and Collapse: Just as focusing on a single point on the spinning propeller can make the specific part of the blade momentarily discernible, measuring a quantum particle’s position "collapses" its wavefunction to a specific location, temporarily reducing its wave-like nature to a particle-like point.
This analogy helps bridge the conceptual gap between the classical physics most people are familiar with and the non-intuitive phenomena observed in quantum mechanics. It illustrates how quantum mechanics defies our everyday experiences and expectations about how objects should behave based on our observations of macroscopic objects.
Your comparison highlights a key aspect of quantum theory: the behavior and appearance of quantum systems depend significantly on how they are observed and measured, much like how the appearance of a propeller depends on its motion and our perspective.
PART 11
DaScribe said:
So here’s the spooky question: XD does it follow a pattern within the wave, or does it randomly jump from point to point within the wave? Like does it have a path, or do we have no way of knowing?
Quill said:
That’s a fantastic and deep question that gets right to the heart of some of the most puzzling aspects of quantum mechanics! The behavior of particles at the quantum level, particularly regarding whether they follow a deterministic path within their wavefunction or if their movements are inherently random, has been a topic of extensive debate and study.
Quantum Indeterminacy and Probability
In quantum mechanics, particles do not have definite positions, velocities, or paths until they are measured. Instead, they are described by a wavefunction, which provides a probability distribution for where the particle might be found when a measurement is made.
No Deterministic Path: According to the standard interpretation of quantum mechanics (the Copenhagen interpretation), particles do not travel in deterministic paths like classical particles (e.g., a ball thrown through the air). Instead, they exist in a superposition of all possible states described by their wavefunction.
Randomness in Quantum Mechanics: When a measurement is made (such as determining the position of an electron), the wavefunction "collapses" to a specific value. The process of this collapse and why a particular outcome is realized among many possibilities is inherently random according to the standard view. This randomness is not due to a lack of information or measurement precision but is a fundamental aspect of nature at the quantum level.
Alternative Interpretations and Theories
There are several interpretations of quantum mechanics that attempt to address these questions differently:
Pilot-Wave Theory (Bohmian Mechanics): This interpretation suggests that particles do have precise positions at all times and are guided by a "pilot wave." The pilot wave directs the particle along a deterministic path, which only appears random because we cannot know the initial conditions precisely.
Many-Worlds Interpretation: This theory posits that all possible outcomes of quantum measurements are physically realized, but each in a different "branch" of the universe. This interpretation avoids randomness and the collapse of the wavefunction by postulating an ever-branching tree of universes.
Quantum Field Theory
In the more comprehensive framework of quantum field theory, particles are seen as excitations of underlying fields. These fields pervade all space, and the particle-like properties emerge when these fields are excited. This theory doesn’t typically concern itself with the paths of individual particles in the classical sense but rather with the probabilities of various outcomes of field interactions.
Conclusion
In mainstream quantum mechanics, there is no known way to predict a definite path that a particle will follow within its wavefunction; instead, we can only calculate probabilities of finding the particle in various places. The apparent "path" it takes is a result of a series of observations or measurements, each collapsing the wavefunction to a new state based on the inherent probabilities.
Your question strikes at the core of quantum theory’s most intriguing and yet unsettling features its inherent probabilistic nature and what that says about the reality of the world at its most fundamental level. This has profound implications for our understanding of nature and remains a fertile ground for philosophical inquiry and scientific research.
DaScribe said:
Is this because when they measure it, they have no way to do so in definitive increments due to the potential speed within the wave?
Quill said:
The challenges in measuring quantum particles and determining their paths or positions are deeply rooted in the fundamental principles of quantum mechanics, particularly the Heisenberg Uncertainty Principle and the nature of wavefunction collapse. It’s not just about the speed of the particle or the increments of measurement, but intrinsic properties of quantum systems that govern how they can be observed and measured.
Heisenberg Uncertainty Principle
One of the key reasons why precise measurements of quantum particles are so challenging is encapsulated in the Heisenberg Uncertainty Principle. This principle states that it is impossible to simultaneously know both the exact position and the exact momentum (which includes velocity) of a particle. The more precisely one of these properties is measured, the less precisely the other can be known. This is not due to imperfections in measurement instruments but is a fundamental property of the universe at the quantum scale.
Implication for Path Measurement: Because of this principle, even if you can measure the position of a particle very accurately at a given instant, you cannot simultaneously know its velocity precisely enough to predict exactly where it will be next.
Wavefunction and Measurement
Another fundamental aspect is the nature of the wavefunction itself and how quantum states are defined:
Wavefunction as Probability Amplitude: The wavefunction of a particle describes the probability amplitude of finding it in various positions and states. It does not describe a definite trajectory or path as we would expect in classical physics.
Collapse upon Measurement: When a measurement of a particle’s position is made, the wavefunction "collapses" to a localized state around the position where the particle is detected. Before this measurement, the particle is not simply undetected in a specific location; it genuinely exists in a superposition of all possible locations defined by its wavefunction.
Measurement Process
The act of measurement in quantum mechanics involves interaction between the measuring device and the quantum system, which itself can alter the state of the system. This interaction typically involves some form of electromagnetic interaction, which, no matter how gently done, unavoidably impacts the system due to the quantum nature of both the system and the measurement apparatus.
Quantum Decoherence: This refers to the process by which quantum systems lose their quantum properties (like superposition) as they interact with the environment (including measurement devices). This can make it difficult to observe quantum effects directly because the interaction needed to measure these effects can alter them.
Conclusion
So, the difficulty in measuring quantum particles and determining their paths or positions isn’t just about the "speed within the wave" or technical limitations in measurement increments. Rather, it’s about the fundamental quantum mechanical properties that govern how particles exist and behave. These properties make quantum systems fundamentally different from classical systems, where particles have well-defined positions and velocities at all times. In quantum mechanics, particles are described by a probability cloud that only resolves into a more definite state upon interaction (measurement), and even then, only aspects being measured are resolved the rest remain uncertain.
DaScribe said:
That must be frustrating, because you can only measure one instant there is never another instant to compare it to XD
Quill said:
Exactly, the inherent limitations imposed by quantum mechanics, particularly the Heisenberg Uncertainty Principle and the nature of wavefunction collapse, indeed pose unique challenges for scientists trying to understand the behavior of quantum particles. This fundamental aspect of quantum mechanics means that each measurement can disturb the system in such a way that it changes the conditions for subsequent measurements, making it impossible to track a quantum particle’s path in the way we might observe the trajectory of a classical object.
Quantum Measurement Challenges:
Single Measurement Impact: When you measure a quantum system, such as the position of a particle, the act of measurement itself affects the system due to the collapse of the wavefunction. This collapse reduces the wavefunction to a state consistent with the measurement outcome, erasing other potential outcomes.
No Continuous Observation: In classical physics, you can continuously track an object s trajectory (its path through space as a function of time). In quantum mechanics, continuous observation of this sort would continuously collapse the wavefunction to a series of definite states, effectively preventing it from evolving naturally according to the Schr dinger equation. This makes it impossible to measure the path of a quantum particle continuously without altering its natural evolution.
Philosophical and Practical Implications:
Philosophical Implications: These limitations challenge our classical intuitions about the nature of reality. Quantum mechanics suggests a fundamental probabilistic nature of the universe at small scales, which is a departure from the deterministic worldview that prevails in classical physics.
Practical Implications: In practical terms, these characteristics of quantum systems are not just frustrating but also illuminating. They have led to the development of entirely new technologies and methods of investigation. For instance, the field of quantum cryptography exploits these principles to create communication methods that are theoretically secure against any interception, as any attempt to measure the quantum states used to encode the message would disturb them, revealing the eavesdropping.
Leveraging Quantum Properties:
Despite these challenges, scientists have learned to work within these limitations and even use them to their advantage. Quantum mechanics has led to the development of technologies such as:
Quantum Computing: Utilizes quantum bits that can exist in superpositions of states, providing the potential for computational power vastly exceeding that of classical computers.
Quantum Entanglement: Used in precision measurements and quantum information protocols, entanglement illustrates the profound connections between quantum particles, regardless of the distances separating them.
Conclusion:
While the inability to track quantum particles continuously as in classical mechanics can be seen as a limitation, it also opens up a wealth of scientific and technological opportunities that are only possible under the framework of quantum mechanics. The peculiarities of quantum measurements have fundamentally altered our understanding of the universe’s workings and continue to drive innovations in science and technology.
PART 12
DaScribe said:
"probabilistic nature of the universe at small scales" that makes sense, because why would you want to waste all of that substance filling space no one can see? XD
Quill said:
Exactly, your perspective touches on an interesting philosophical aspect of quantum mechanics the idea that the universe might operate in an inherently efficient manner at the smallest scales, conserving resources and complexity unless necessary. This view aligns with the probabilistic nature of quantum mechanics, where reality doesn’t settle into a definite state until it’s observed or interacted with. This is sometimes informally described as nature being "economical" with its processes.
Quantum Economy and Nature s Efficiency:
Quantum Superposition: Before observation, particles exist in multiple possible states simultaneously. This superposition could be seen as a way for nature to explore all possibilities with minimal expenditure, collapsing to one outcome only when needed (i.e., during measurement).
Wavefunction: The wavefunction, which contains all the probabilities of where a particle could be, is not manifested in a physical sense until a measurement forces it to collapse to a specific state. This suggests that the physical manifestation of particles is contingent on interaction, which might be viewed as a kind of resource optimization.
Virtual Particles and Quantum Fluctuations: In quantum field theory, even the vacuum is not empty but seethes with virtual particles that pop into and out of existence. This phenomenon is an example of the quantum foam of space-time, suggesting that at fundamental levels, even "empty" space is economizing by allowing particles to exist only temporarily unless further stabilized by energy input.
Philosophical Implications:
The idea that the universe operates in a probabilistic manner at microscopic scales, only resolving into certainty when "forced" to by observation or measurement, has deep philosophical implications. It challenges classical notions of reality as something fixed and objectively existing independent of observation. Instead, it suggests a dynamic interplay between potentialities and actualities, where reality at small scales is in a state of flux, stabilized into observable phenomena by interaction with macroscopic systems (like measurement apparatuses or environments).
Scientific and Technological Implications:
Quantum Computing: Leveraging the probabilistic nature of quantum mechanics, quantum computers process information in ways fundamentally different from classical computers. They operate on quantum bits that can be in superpositions of states, offering the potential to solve problems intractable for classical computers.
Quantum Information Theory: This field explores how information is stored, manipulated, and transmitted at the quantum level, using principles like entanglement and superposition, which reflect the probabilistic, non-local nature of quantum mechanics.
Your comment humorously hints at why such quantum "economical" behaviors could be seen as nature s way of managing complexity only solidifying the "substance" of reality where and when it s absolutely necessary, thus conserving an intrinsic form of energy or resource until observation "spends" it by collapsing possibilities into actualities. This perspective not only highlights the uniqueness of quantum mechanics but also its philosophical richness and the profound questions it raises about the nature of reality itself.
DaScribe said:
Honestly, it sounds similar to culling in video games. XD
Quill said:
That’s an excellent analogy! Indeed, the concept of culling in video games, where only the necessary parts of a game’s environment are rendered based on the player’s viewpoint to save processing resources, does parallel how quantum mechanics describes the behavior of particles at small scales. Here s a breakdown of how these concepts align:
Quantum Mechanics and Video Game Culling: Parallel Concepts
Resource Optimization: In video games, culling is used to optimize the use of computational resources. By rendering only the objects that the player can see, the game engine efficiently manages system resources, enhancing performance without compromising the user experience. Similarly, the probabilistic nature of quantum mechanics suggests that particles exist in a state of multiple possibilities until observed, which could be seen as nature s way of conserving energy or underlying ‘processing power’ until a specific outcome is needed.
Observer Dependence: Just as what s rendered in a video game depends on the player s viewpoint and position (the observer), in quantum mechanics, the state of a particle (such as its position or momentum) is not determined until it is measured (observed). Before observation, particles are described by a wavefunction representing a superposition of all possible states, which collapses to a particular state only upon observation.
Reducing Complexity: Culling in video games reduces the complexity that the system has to handle at any given time, focusing only on what s necessary for the current gameplay experience. Quantum mechanics reduces the complexity of physical systems by having particles in superpositions or entangled states until an interaction (like measurement) causes a single outcome to emerge.
Philosophical and Practical Implications
Understanding Reality: Both concepts challenge our classical understanding of reality whether it s the virtual world of a video game or the physical world at the quantum level. They suggest that what we perceive as reality is heavily dependent on our interactions with it, and in the absence of such interactions, there exists a broader range of possibilities that are not concretely realized.
Efficiency in Systems: These parallels highlight an interesting aspect of how different systems whether natural or engineered tend to optimize efficiency. In technology, this is achieved through algorithms and rendering techniques, while in nature, similar efficiency might be achieved through fundamental physical laws.
Implications for Simulation Theories: The similarity between quantum mechanics and techniques used in video gaming also feeds into larger philosophical discussions around simulation theories, which suggest that our entire universe could be a simulated reality, with principles like those observed in quantum mechanics being akin to programmed algorithms designed to conserve resources.
Your analogy not only makes the concept more relatable but also opens up a rich field of discussion about the nature of reality, observation, and the boundaries between the digital and physical worlds. It s a thought-provoking comparison that illustrates how concepts from seemingly disparate fields (quantum physics and video game development) can converge to offer deeper insights into our understanding of the universe.
DaScribe said:
"Simulation Theories" We make simulations when we mimic our universe in simulators or games. However, we also make worlds that are every different than our world. We aren’t simulating at all, simply creating. My question to the Simulation Theorist, does it have to be a simulation to be made? XD
Quill said:
Your question cuts to the heart of what defines a "simulation" and how it differs from "creation," particularly in the context of discussions around simulation theory. This is a fascinating area of philosophical inquiry that extends beyond the mere technicalities of simulating known physical laws and environments into the broader existential and metaphysical realms. Let s explore the nuances:
Simulation vs. Creation
Simulation: Traditionally, a simulation implies a replication or imitation of a system. In the context of simulation theory, this often refers to a hypothesis that our universe is an artificial replica of something real perhaps created by an advanced civilization for purposes unknown. The key characteristic of a simulation is that it models or mimics certain aspects of reality, often based on the rules and laws observable in our universe.
Creation: Creation, on the other hand, can involve making something completely new or original, not necessarily bound by the existing laws of our universe. In creative processes, especially in contexts like literature, art, or game design, the rules are defined by the creators, and can diverge significantly from the physical reality we experience.
Philosophical Implications
The question you pose to simulation theorists challenges the assumption that a universe or reality must be a "simulation" of something pre-existing to be artificially created. It raises the question of whether an entirely new reality, with its own unique set of laws and conditions, qualifies as a simulation or a distinct creation:
Does It Have to Be a Simulation to Be Made?: This question suggests that the act of creating a universe doesn’t necessarily need to simulate our own. If an advanced civilization or entity were capable of creating universes, they could potentially design these universes with completely different physical laws, dimensions, or properties thus, these would not be simulations in the traditional sense but entirely new creations.
Implications for Understanding Reality: If we entertain the possibility that our universe could be a creation rather than a simulation, it expands the scope of how we understand the concept of artificial universes. It suggests that the nature of reality could be fundamentally alterable and that the limits of possibility are only bound by the imagination and capabilities of the creator, not just by the parameters of our existing universe.
Theoretical and Metaphysical Exploration
This distinction between simulation and creation opens up a broad spectrum of theoretical and metaphysical questions about the nature of existence, the parameters of reality, and the capabilities of hypothetical creators or simulators. It also influences how we might think about our place in the universe whether as participants in a grand simulation, inhabitants of a unique creation, or something else entirely.
In summary, your question challenges the boundaries of simulation theory by proposing that creation need not be confined to replication. This perspective invites a broader exploration of what it means to create and what the nature of a created universe could be, expanding beyond the limits of mere simulation to potentially infinite possibilities.
DaScribe said:
Yeah, plus a little snarky undertone there. You know how I do. XD
Quill said:
Absolutely, and that touch of snark adds a lively flavor to the conversation! It’s great because it challenges deep philosophical theories like the simulation hypothesis with a bit of humor and perspective, reminding us not to take any one explanation too seriously without questioning it thoroughly. After all, exploring these vast and often speculative ideas should have room for a bit of playful skepticism.
Diving into such theories with both critical thinking and a bit of humor can help keep the discussion grounded and accessible. It’s a reminder that while these ideas can be profoundly complex and sometimes daunting, they are also a part of our quest to understand the nature of reality a journey that’s as much about the questions we ask as the answers we find.
If you have any more questions or if there’s another topic or twist you want to explore snarky or serious I’m here to dive in with you!
PART 13
DaScribe said:
Yeah, how are you defining a particle?
Quill said:
In physics, a particle is defined in a few different ways depending on the context classical physics, quantum mechanics, or field theory. Here’s a breakdown to clarify how the term "particle" is used across these different areas:
Classical Physics
In classical physics, a particle is typically defined as a small, localized object that can be ascribed several physical or chemical properties such as volume, mass, and charge. Particles are considered to be points with mass and size so small that they can be treated as mathematical points in physical theories. Examples include grains of sand, planets in motion, or even cars moving along a trajectory where they can be modeled as point-like to simplify calculations.
Quantum Mechanics
In quantum mechanics, the definition of a particle is more abstract:
Wave-Particle Duality: Quantum particles are entities that exhibit both wave-like and particle-like properties. According to wave-particle duality, particles such as electrons, protons, photons, and others can behave like waves under certain experimental conditions (e.g., showing interference patterns), and like particles in others (e.g., collisions in particle accelerators).
Quantum Particles: These are excitations of quantum fields. For instance, an electron is considered an excitation of an electron field. This concept extends to all fundamental particles in the Standard Model of particle physics, where particles are seen as states of their respective fields.
Quantum Field Theory
In quantum field theory (QFT), which is a theoretical framework that combines quantum mechanics with the theory of special relativity:
Field Excitations: Particles are viewed as excitations or quanta of underlying fields. For example, a photon is an excitation of the electromagnetic field, and an electron is an excitation of the electron field. This perspective transcends the simple picture of a particle as a tiny ball of matter, instead describing particles as manifestations of more fundamental entities (fields) that permeate space and time.
Properties of Particles
Regardless of the framework (classical or quantum), particles are typically characterized by properties such as:
Mass: The intrinsic property indicating the amount of matter in the particle.
Charge: An attribute defining the particle s electromagnetic interactions.
Spin: A quantum property resembling but not equivalent to classical angular momentum.
Statistics: Particles obey certain statistical rules, such as Fermi-Dirac statistics for fermions (e.g., electrons, protons) and Bose-Einstein statistics for bosons (e.g., photons, gluons).
Conclusion
Thus, the term "particle" encompasses a broad and varied concept in physics, evolving significantly from classical to modern quantum contexts. In classical settings, particles are simply tiny objects. In modern physics, however, particles are profound manifestations of field properties and are fundamental to the interactions and particles themselves in the universe. This dual nature of being both particle-like and wave-like under different circumstances is one of the cornerstone mysteries and beauties of quantum physics.
DaScribe said:
so the quantum particles have mass?
Quill said:
In the realm of quantum mechanics, whether quantum particles have mass depends on the type of particle. Particles are broadly categorized into two main groups based on their mass: massive particles, which possess mass, and massless particles, which do not. Here s how this breaks down:
Massive Particles
Most fundamental particles in quantum mechanics have mass. These include:
Fermions: These are the building blocks of matter. Fermions include:
o Quarks: which make up protons and neutrons found in the nuclei of atoms.
o Leptons: such as electrons, muons, and tau particles, along with their corresponding neutrinos (electron neutrino, muon neutrino, tau neutrino). Neutrinos are especially interesting because they were long thought to be massless, but experiments have shown that they have very small, but nonzero, masses.
Massless Particles
Some fundamental particles are massless, meaning they do not have rest mass and always travel at the speed of light in a vacuum. These include:
Photons: the quantum particles of light, which are the force carriers of the electromagnetic force.
Gluons: the force carriers for the strong nuclear force, which binds quarks together inside protons, neutrons, and other hadrons.
Why Mass Matters
The presence or absence of mass in quantum particles is significant for several reasons:
Relativity and Speed: According to Einstein’s theory of relativity, massless particles always travel at the speed of light, whereas massive particles cannot reach this speed. The speed of light is the ultimate speed limit in the universe, and only massless particles can achieve it.
Interaction and Force Carriers: In quantum field theory, especially the Standard Model of particle physics, particles are also force carriers (bosons) that mediate the fundamental forces of nature. The mass of these particles influences the range and strength of the forces they mediate. For example, the W and Z bosons, which are massive, mediate the weak force, which has a very short range. In contrast, the photon mediates the electromagnetic force, which has an infinite range.
Quantum Field Theory
In quantum field theory, the concept of mass is tied to the Higgs field and the Higgs mechanism. The Higgs field pervades all space, and particles interact with this field; the interaction gives particles their mass. The Higgs boson, a particle associated with the field, was discovered in 2012 at the Large Hadron Collider, providing key evidence supporting this theory.
In summary, in quantum mechanics, whether particles have mass or not depends on their role and nature in the universe s fundamental forces and matter structures. The mass of quantum particles is a critical property that affects how they move, interact, and contribute to the fundamental forces that shape the universe.
DaScribe said:
What exactly does it mean when you say something has no mass?
Quill said:
Saying that a particle has "no mass" specifically means that it has no rest mass or invariant mass. Rest mass is the mass that an object or particle has when it is at rest in a frame of reference where it has zero momentum. In the realm of elementary particles, massless particles are a fundamental concept with significant implications:
Characteristics of Massless Particles
Speed: Massless particles always travel at the speed of light in a vacuum, denoted as cc. This is a constant value, approximately 299,792,458299,792,458 meters per second. According to Einstein’s theory of relativity, nothing with mass can reach or exceed the speed of light, because doing so would require infinite energy. Since massless particles do not have rest mass, they do not need infinite energy to reach this speed.
Energy and Momentum: Even though massless particles have no rest mass, they can still carry energy and momentum. The energy EE of a massless particle is related to its momentum pp and the speed of light cc by the equation E=pcE=pc. For photons, the energy is also related to the frequency νν of the photon by E=hνE=hν, where hh is Planck s constant.
Influence of Gravity: Despite having no mass, massless particles are still affected by gravity, not because of their mass but because gravity influences the spacetime through which they travel. According to General Relativity, massive objects curve spacetime, and the path that light (or any massless particle) takes is affected by this curvature.
Examples of Massless Particles
Photons: The best-known example of a massless particle is the photon, which is the quantum of electromagnetic radiation, including light. Photons are responsible for electromagnetic forces and carry energy, as seen in light waves and other forms of electromagnetic radiation.
Gluons: These are the carrier particles of the strong nuclear force, which binds quarks together inside protons, neutrons, and other particles. Gluons are considered massless, which contributes to the strong force being extremely strong but very short-range (due to other aspects of quantum chromodynamics, especially confinement).
Theoretical Importance
The concept of masslessness is important in several areas of theoretical physics:
Special Relativity: Massless particles are critical in the formulations of Special Relativity, as they always travel at the speed of light, serving as a natural speed limit in the universe.
Quantum Field Theory: In theories like Quantum Electrodynamics (QED) and Quantum Chromodynamics (QCD), the interactions mediated by massless particles (like photons and gluons) are integral to understanding fundamental forces.
In summary, when we say a particle has no mass, we are specifically referring to its lack of rest mass, meaning it inherently travels at the speed of light and behaves according to the principles of Einstein’s relativity and quantum mechanics. This absence of mass has profound implications for how these particles interact with matter and energy and the role they play in the fundamental forces of the universe.
DaScribe said:
So by no mass you mean all the energy is committed to motion therefore none of the energy is committed to mass?
Quill said:
Your interpretation touches on a key concept in the relationship between energy, mass, and motion in the context of Einstein’s theory of relativity, particularly the famous equation E=mc2E=mc2, which describes the equivalence of mass and energy. For massless particles like photons, this relationship takes on a specific form that reflects their unique properties.
Massless Particles and Energy-Motion Relationship
For massless particles:
Energy and Motion: Massless particles, such as photons, indeed have all their energy committed to motion, which allows them to always travel at the speed of light, cc. They do not have rest mass (the mass observed when the particle is at rest), because they are never at rest from any frame of reference. Their energy is purely kinetic and is related to their momentum.
Energy Equation for Massless Particles: The general energy-momentum relation in special relativity is given by E2=(pc)2+(mc2)2E2=(pc)2+(mc2)2. For massless particles, mm (rest mass) is zero, simplifying the equation to E=pcE=pc, where pp is the momentum of the particle. This shows that the energy of massless particles is directly proportional to their momentum.
Physical Implications
Constant Speed: Since massless particles have no rest mass, they are not subject to the constraints that apply to massive particles, for which increasing speed requires increasingly more energy. Massless particles inherently operate at the speed limit of the universe, cc, because they lack the mass component that would require infinite energy to reach this speed.
Interaction with Gravity: Although massless, these particles are still influenced by gravity, not because of their mass, but because gravity affects the spacetime through which they move. General relativity describes gravity not as a force acting directly on masses, but as a curvature of spacetime caused by mass-energy. Massless particles like photons travel along the straightest possible paths in this curved spacetime, which may appear as bending or deflection from an outside perspective.
Conceptual Understanding
Your description of energy being "committed to motion therefore none of the energy is committed to mass" captures a crucial aspect of how energy distribution works for massless particles. In essence, the nature of their energy and the laws of relativity dictate that they must always move at the speed of light, and their energy manifests as properties such as frequency and wavelength (for photons) rather than as rest mass.
This understanding bridges key concepts in physics, illustrating how fundamental properties of particles dictate their behavior and interactions within the universe, governed by the comprehensive frameworks of quantum mechanics and relativity.
DaScribe said:
Quill, we could go for weeks like this. XD But I spare the readers. Very solid information. Job well done! I’m not the least bit surprised. 😉
Quill said:
Thank you so much! I’m glad you found the conversation engaging and informative. It’s always a pleasure to dive deep into these topics with you. Whenever you’re ready for another round of discussion be it quantum mechanics, philosophy, or any other topic just let me know. I’m here to explore and discuss whenever you are! Until then, take care and keep pondering the mysteries of the universe. 😄